Lanthanum(III) bromide
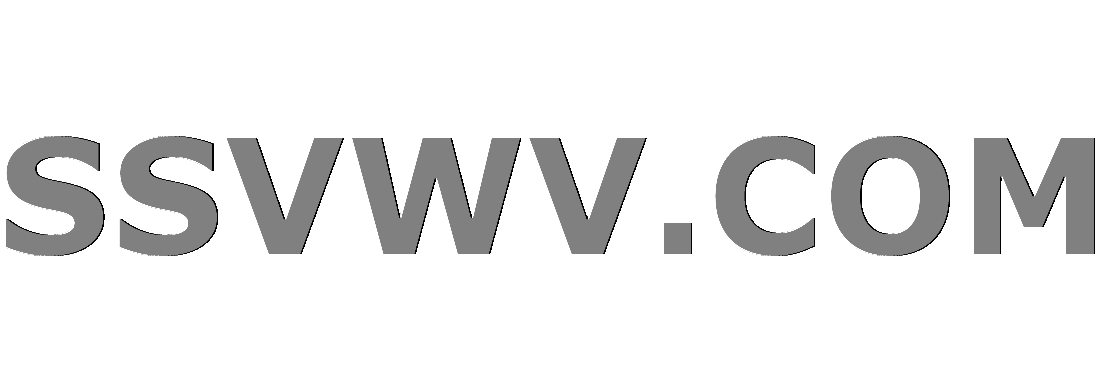
Multi tool use
| |||
Names | |||
---|---|---|---|
IUPAC names Lanthanum(III) bromide Lanthanum tribromide | |||
Identifiers | |||
CAS Number |
|
||
3D model (JSmol) |
|
||
ChemSpider |
|
||
ECHA InfoCard |
100.033.527 |
||
EC Number |
36-896-7 |
||
PubChem CID |
|
||
InChI
| |||
SMILES
| |||
Properties | |||
Chemical formula |
LaBr3 |
||
Molar mass |
378.62 g/mol (anhydrous) |
||
Appearance |
white solid, hygroscopic |
||
Density |
5.06 g/cm3, solid |
||
Melting point |
783 °C (1,441 °F; 1,056 K) |
||
Boiling point |
1,577 °C (2,871 °F; 1,850 K) |
||
Solubility in water |
Very soluble |
||
Structure | |||
Crystal structure |
hexagonal (UCl3 type), hP8 |
||
Space group |
P63/m, No. 176 |
||
Coordination geometry |
Tricapped trigonal prismatic (nine-coordinate) |
||
Hazards | |||
EU classification (DSD) (outdated) |
not listed |
||
Flash point |
not flammable |
||
Related compounds | |||
Other anions |
Lanthanum(III) fluoride Lanthanum(III) chloride Lanthanum(III) iodide |
||
Other cations |
Cerium(III) bromide Praseodymium(III) bromide |
||
Except where otherwise noted, data are given for materials in their standard state (at 25 °C [77 °F], 100 kPa). | |||
![]() ![]() ![]() | |||
Infobox references | |||
Lanthanum(III) bromide (LaBr3) is an inorganic halide salt of lanthanum. When pure, it is a colorless white powder. The single crystals of LaBr3 are hexagonal crystals with melting point of 783 °C. It is highly hygroscopic and water-soluble. There are several hydrates, La3Br·x H2O, of the salt also known. It is often used as a source of lanthanum in chemical synthesis and as a scintillation material in certain applications.
Lanthanum bromide scintillation detector
The scintillator material cerium activated lanthanum bromide (LaBr3:Ce) was first produced in 2001.[1] LaBr3:Ce-based radiation detectors offer improved energy resolution, fast emission and excellent temperature and linearity characteristics. Typical energy resolution at 662 keV is 3% as compared to sodium iodide detectors at 7%.[2] The improved resolution is due to a photoelectron yield that is 160% greater than is achieved with sodium iodide. Another advantage of LaBr3:Ce is the nearly flat photo emission over a 70 °C temperature range (~1% change in light output)[citation needed].
Today LaBr3 detectors are offered with bialkali photomultiplier tubes (PMT) that can be two inches in diameter and 10 or more inches long[citation needed] . However, miniature packaging can be obtained by the use of a silicon drift detector (SDD) or a Silicon Photomultiplier (SiPM).[3] These UV enhanced diodes provide excellent wavelength matching to the 380 nm emission of LaBr3. The SDD is not as sensitive to temperature and bias drift as PMT. The reported spectroscopy performance of the SDD configuration resulted in a 2.8% energy resolution at 662 keV for the detector sizes considered.
LaBr3 introduces an enhanced set of capabilities to a range of gamma spectroscopy radioisotope detection and identification systems used in the homeland security market. Isotope identification utilizes several techniques (known as algorithms) which rely on the detector’s ability to discriminate peaks. The improvements in resolution allow more accurate peak discrimination in ranges where isotopes often have many overlapping peaks. This leads to better isotope classification. Screening of all types (pedestrians, cargo, conveyor belts, shipping containers, vehicles, etc.) often requires accurate isotopic identification to differentiate concerning materials from non-concerning materials (medical isotopes in patients, naturally occurring radioactive materials, etc.) Heavy R&D and deployment of instruments utilizing LaBr3 is expected in the upcoming years.
References
^ Van Loef, E. V. D; Dorenbos, P; Van Eijk, C. W. E; Krämer, K; Güdel, H. U (2001). "High-energy-resolution scintillator: Ce3+ activated LaBr3". Applied Physics Letters. 79 (10): 1573–1575. doi:10.1063/1.1385342..mw-parser-output cite.citation{font-style:inherit}.mw-parser-output q{quotes:"""""""'""'"}.mw-parser-output code.cs1-code{color:inherit;background:inherit;border:inherit;padding:inherit}.mw-parser-output .cs1-lock-free a{background:url("//upload.wikimedia.org/wikipedia/commons/thumb/6/65/Lock-green.svg/9px-Lock-green.svg.png")no-repeat;background-position:right .1em center}.mw-parser-output .cs1-lock-limited a,.mw-parser-output .cs1-lock-registration a{background:url("//upload.wikimedia.org/wikipedia/commons/thumb/d/d6/Lock-gray-alt-2.svg/9px-Lock-gray-alt-2.svg.png")no-repeat;background-position:right .1em center}.mw-parser-output .cs1-lock-subscription a{background:url("//upload.wikimedia.org/wikipedia/commons/thumb/a/aa/Lock-red-alt-2.svg/9px-Lock-red-alt-2.svg.png")no-repeat;background-position:right .1em center}.mw-parser-output .cs1-subscription,.mw-parser-output .cs1-registration{color:#555}.mw-parser-output .cs1-subscription span,.mw-parser-output .cs1-registration span{border-bottom:1px dotted;cursor:help}.mw-parser-output .cs1-hidden-error{display:none;font-size:100%}.mw-parser-output .cs1-visible-error{font-size:100%}.mw-parser-output .cs1-subscription,.mw-parser-output .cs1-registration,.mw-parser-output .cs1-format{font-size:95%}.mw-parser-output .cs1-kern-left,.mw-parser-output .cs1-kern-wl-left{padding-left:0.2em}.mw-parser-output .cs1-kern-right,.mw-parser-output .cs1-kern-wl-right{padding-right:0.2em}
^ Knoll, Glenn F., Radiation Detection and Measurement 3rd ed. (Wiley, New York, 2000).
^ A. Dawood Butt et al., "Comparison of SiPM and SDD based readouts of 1″ LaBr3:Ce scintillator for nuclear physics applications," 2015 IEEE Nuclear Science Symposium and Medical Imaging Conference (NSS/MIC), San Diego, CA, 2015, pp. 1-4.
doi: 10.1109/NSSMIC.2015.7581734
WV W1sGFGWcY4y1A